It’s no secret that the peels and skins of produce (think sweet potatoes, apples, tomatoes) contain a majority of the food’s nutritional value. But citrus pith is among the most concentrated sources of many beneficial nutrients–there are over 60 different flavinoids in the fruits in general–which makes it an ideal way to amp up your healthfulness (especially during the winter, when your immunity needs a boost) without adding anything to your diet. If you can’t handle the taste or texture of the pith on its own, try adding them to a smoothie or adding zest to sauces, dressings, and other recipes (some lemon zest can give a nice zing to quinoa pilaf or a tofu glaze).

Start at the top, just where the pith (white part) meets the pulp and slice off the skin, following the curve of the fruit.
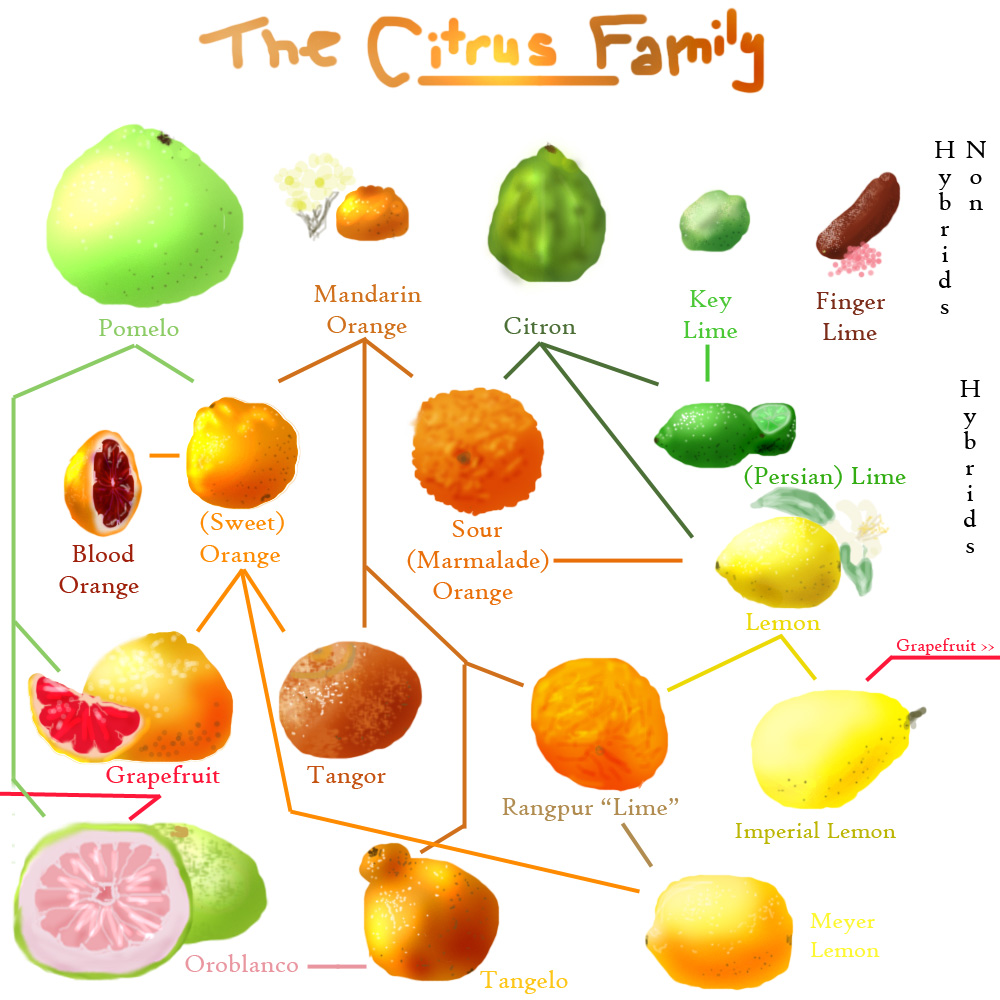
Grapefruits, mandarin, and lemons (just the peel) contain naringenin, which can stimulate the liver to burn excess fat and reduce blood sugar, triglycerides, and cholesterol; it’s such a powerful antioxidant that it can reverse the DNA damage that causes cancer and radiation-induced damage to the cells of the body. Lemons, limes, grapefruit and oranges, contain hesperidin, which studies show can reduce bone loss and lipids post-menopause. The compound that gives citrus its smell, d-limonene, likewise fights cancer, high cholesterol, and indigestion from stomach acid.
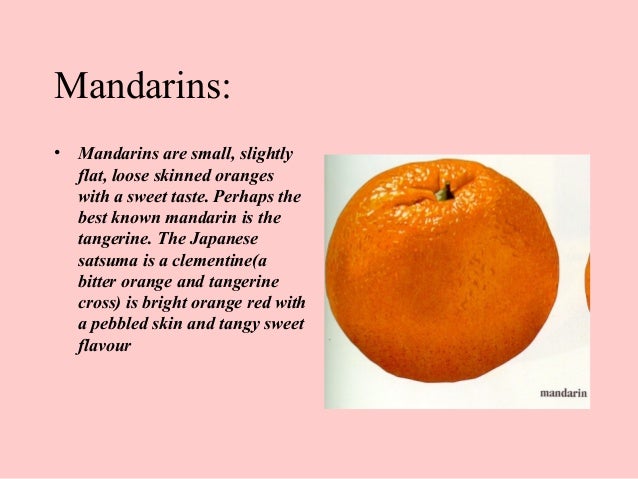
Mandarins are small, slightly flat, loose skinned oranges with a sweet taste. Perhaps the best known mandarin is the tangerine. The Japanese Satsuma is a clementine (a bitter orange and tangerine cross) is bright orange red with a pebbled skin and tangy sweet flavor.
Citrus’s claim to fame, vitamin C, is also more potent in the peel: compared to one wedge of lemon, 1 T. of peel as twice as much vitamin C and three times as much fiber. You’ll also find vitamins A, C, B6 and B5; calcium; riboflavin; thiamin; niacin; and folate.
An added bonus is that consuming citrus pith means reducing your food waste. A win-win for body and planet!

Schematic diagram of chromoplast differentiation in the flesh of citrus fruits. A to E.



When you peel an orange, don't throw away the white part under the skin: Bioflavonoids help fight cancer and obesity.

Citrus fruits have long had a reputation for high concentrations of healthful nutrients such as vitamin C, and recent research continues to uncover new health benefits from many citrus flavonoids. Although the flavonoids are abundant within the fruit itself, they are found in highest concentration in the peel -- which means that unless you're cooking with citrus peel or zest, the best way to get the full antioxidant punch is to eat a bit of the tart, white flesh on the inside of the fruit's peel.
One of the most promising of these flavonoids is naringenin, which occurs in all citrus fruits and in tomato peel. Studies have shown that naringenin and related citrus flavonoids can repair the DNA damage that leads to cancer, and other research has shown that the phytonutrient can stimulate the liver to burn excess fat and restore obese mice to a normal weight.
Naringenin has also restored normal levels of triglyceride and cholesterol levels in animal studies, as well as restoring healthy blood sugar levels. There's medicine in your food! So don't throw that medicine away with the peel. Eat it and enjoy the many health benefits offered by Mother Nature.
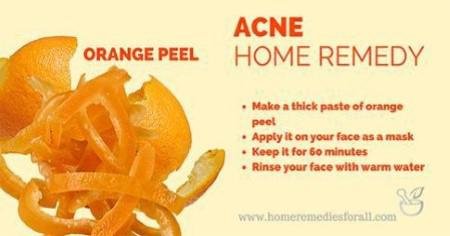
ACNE HOME REMEDY USE ORANGE PEEL.
Make a thick paste of orange peel.
Apply it on your face as a mask.
Keep it for 60 minutes.
Rinse your face with warm water.
THE INVESTIGATION OF CITRUS FRUIT QUALITY. POPULAR CHARACTERISTIC AND BREEDING
Abouzar Abouzari1, Nafi seh Mahdi Nezhad21 Agricultural Research and Education Center, Mazandaran, Iran
Department of Plant Breeding and Biotechnology, Faculty of Agriculture, University of Zabol, Iran
Abstract ABOUZARI ABOUZAR, MAHDI NEZHAD NAFISEH. 2016.
The Investigation of Citrus Fruit Quality.
Popular Characteristic and Breeding. Acta Universitatis Agriculturae et Silviculturae Mendelianae Brunensis, 64(3): 725–740.
Citrus is the most economically important fruit crop in the world. In citrus, the concept of fruit quality comprises several other aspects intimately related to human health apart from physical attributes and diet components. Citrus is an excellent model to study fruit quality because of its peculiar fruiting, singular biochemistry and economical relevance. A citrus breeding programme starts with the selection of suitable parents and the planning of controlled crosses. Information on the breeding value of available parents and the heritability of specific characters is important in a plant breeding programme to aid the breeder in parent selection and the planning of controlled crosses. Major goals of variety breeding in citrus are mostly related to fruit quality, productivity and harvesting period. In a broad sense, citrus fruit quality includes many physical attributes like fruit color, fruit size, easy of peeling and seedlessness. These traits have become paramount in commercial citrus types and new cultivar being developed through plant breeding and selection of new sports. This paper focus on four main citrus characteristics that responsible for fruit quality and are the basis for judging the product acceptability by consumers. We also discuss the variety strategy for citrus quality improvement.
Keywords: citrus, fruit quality, plant breeding
INTRODUCTION
The term citrus originated from the Latin form of ‘Kedros’ a Greek word denoting trees like cedar, pine, and cypress. As the smell of citrus leaves and fruit is reminiscent of that of cedar, the name citrus has been applied to the citron. Linnaeus grouped all citrus species known to him in the genus Citrus. In Greek mythology citrus fruits are called hesperides. The suggested origin of the true citrus fruits is South East Asia, including South China, north-eastern India and Burma. Evidence from wild citrus in the area is still unclear. In many cases, seed has been spread long distances from the sites of origin and culture by birds, water streams, and human activity (Spiegel-Roy et al., 1996). The genus Citrus belongs to the subtribe Citrinae, tribe Citreaea, subfamily Aurantioideae of the family Rutaceae. This genus may be further divided into two subgenera (Citrus and Papeda), based on leaf, flower, and fruit properties. Citrus species are essentially diploids (2n = 2x = 18). On the basis of morphological characteristics, studies have been carried out on the relationships between genera and species (Jain and Pryadarshan, 2009). This has led to the formulation of numerous classification systems. The most commonly used citrus classifications are by Swingle (Swingle and Reece, 1967) and Tanaka (Tanaka, 1977). In the genus Citrus, Swingle recognized only 16 species, whereas Tanaka recognized 162 species (Tadeo et al., 2008). The difference in these two systems reflected opposing theories on what degree of morphological difference justified species status and whether presumed hybrids among naturally occurring forms should be given species status. With a total world production of 149.431 million tons, citrus is one of the world’s most important fruit crops (FAO, 2015). Its importance to agriculture and the world’s economy is demonstrated by its wide distribution and large-scale production (Soost and Roose, 1996).
There has been a strong international market demand for easy peeling type citrus fruit in recent years. These include seedless clementines in the US(United States) and EU (European Union) and satsuma mandarins in Asia and the U.K. The seedless and easy peeling characteristics of these types of citrus fruit provide a market advantage over traditional seeded type citrus. The world grapefruit market has shi ed signifi cantly toward seedless high-pigmented colored types. The US and EU market almost exclusively requires cultivars with a red pulp high in carotenoids. The Japanese market is another traditionally strong grapefruit market that is shi ing to pigmented grapefruit, although the non-pigmented types still have a high demand. Other quality parameters important in international marketing of citrus fruit include peel fi rmness and juice content in lemons and limes. Depending on the needs of a specifi c region of the world, the objectives of citrus breeding programs may vary considerably. For example, some of the objectives may have high priority in some regions and low or no priority in others. However, breeding for quality is one of the important objectives in most regions (Khan, 2007).Citrus Fruit QualityQuality is a term that when applied to fresh fruit may convey a number of interpretations. Usually within the markets it refers to the external appearance of the product. Within the EU and the US, quality standards are applied to fresh fruit and vegetables. And products exported to them from countries outside Europe and the US must conform to essential standards. In these standards, fruit and vegetables according to their grade (i.e., extra, fi rst, and second) are packed and graded so as to be virtually free of injury, blemishes, and diseases and to be uniform in shape, size, color, and maturity. Quality, however, does not only relate to appearance, but also some other characterization for example: taste, rich in nutrients, vitamins, seedlessness, odour, etc. must be considered (Jenks and Bebeli, 2011). Irrespective of the yielding potential of a newly developed cultivar, success in agriculture will be determined by the end-use quality of the saleable product. There are two main types of end-use quality: Organoleptic – consumer acceptance or preference of taste, size, texture, and colour. Although many people diff er in their preference, there is o en agreement within taste panels as to general preference towards certain levels of expression of these attributes, thus ‘liking’ some genotypes over others (even disregarding ‘off -tastes’). Chemical – where quality is determined by chemical analyses of the harvestable product. This is perhaps easily understandable in saying, oil crops, where the quality of the oil can be determined with great accuracy by the determination of the oil fatty acid profi le, or in the pharmaceutical (or farm-aceutical) industry where the quality of drugs are chemically determined. However, these are also true for fi bre plants like cotton, and indeed fodder crops where protein content and digestibility are determined by analytical methods (Brown and Caligari, 2011). Overall, quality is what creates the demand for a product. It is the end-user who will mostly determine if that crop will be grown in future years. It is a very naïve breeder who ignores the fact that consumer preference is continually changing and that the quality standards of today may be superseded by a newset of standards in the future. It is, therefore, imperative to organize a breeding scheme to be fl exible and to try to cover as many potential aspects of yield, quality, and other factors which may be important in the next two decades (Brown and Caligari, 2011).The objectives of citrus fruit production and the methods applied to achieve it are diff erent depending on the fi nal destination of fruit, that is, the processing industry or the fresh market (Davies and Albrigo, 1994). The most appreciated features of citrus fruit for processing are related to internal fruit quality which is a function of fl avour and palatability. Citrus fruit for processing must have no decay symptoms, elevated juice content, high level of TSS (total soluble sugars, mostly sucrose, glucose and fructose), low TA (titratable acidity, mostly citric acid) and minor amounts of bitter components such as the triterpene limonin and the fl avonoid naringin. The external appearance of fruits, however, is not particularly important for processing although attractive internal colour in oranges is usually required. Citrus fresh fruit quality standards, on the other hand, are largely dependent on consumers’ preferences that in addition may change substantially between countries. In general, considerable more emphasis is put in fresh markets on external than on internal fruit quality standards. Although citrus fruit must reach a minimum ‘maturity index’ (the TSS to TA ratio; in general, 7–9 for oranges and mandarins and 5–7 for grapefruits) to be marketable, the essential quality requirements of citrus fruit for fresh market are mostly cultivar characteristics such as fruit size and shape, brightly coloured and fi rm rind, and absence of surface blemishes (Tadeo et al., 2008).Mandarins, navel oranges, some common oranges, pummelos, and grapefruits are mainly for fresh markets; lemons are for both fresh and juice markets. Generally, both external and internal fruit quality are equally important in the fresh market. Suitable fruit shape, deep peel color, and smooth and shiny appearance are the most attractive external quality characteristics for the consumers. Easy peeling, pleasant fl avor, seedlessness, and fragrance are the most desired internal quality parameters (Jenks and Bebeli, 2011).Breeding ObjectivesObjectives are the fi rst of the plant breeder’s decisions. Breeding objectives are manifold and vary from hardy cultivars, adapted to particular climatic and soil conditions, improved tree vigour and
The Investigation of Citrus Fruit Quality. Popular Characteristic and Breeding 727longevity, reduced tree size, fruit quality, industrial purposes, season of ripening and to disease and pest resistance. The reasons for the low impact of traditional breeding of this major fruit crop are related to the peculiarities of citrus reproductive biology so the plant breeder for fully utilizing the variability in citrus, faced with some barriers include: the incompatibility, sterility and poly-embryony, facultative parthenocarpy, heterozygosity, and the prolonged juvenile period that occur in some varieties. In addition to this unusual reproductive biology, citrus also possess a rare combination of intriguing biological characteristics including non-climacteric fruit ripening, juvenility, dormancy, surprising root/shoot interactions, and several other specifi c tree traits. Overall, variety development is extremely slow and inherently costly because it requires many years and eff orts to adequately evaluate fruit quality improvement. Furthermore, many of the commercial citrus types produce polyembryonic seeds through nucellar embryony. These circumstances and the narrow germplasm bases actually represented in citrus have precluded breeding as a strategy for cultivar development and improvement (Iglesias et al., 2009).Breeding eff orts for the improvement of citrus fruit quality have been focused on traits that fi rst attribute perceived by the consumers and make fruit more diffi cult to eat: peel and pulp color, fragrance, pulp taste, seedlessness, improvement of fruit size and easy peeling. Thus, we want a fruit that is seedless, can be eaten without peeling, is bite size, and does not spurt juice out when eaten. Hundreds of cultivars have been released to meet diff erent market requirements.Fruit ColorColor is the most important quality characteristic of fruits because it is the fi rst attribute perceived by the consumers and is the basis for judging the product acceptability. The most important color changes in fruits are related to chemical, biochemical, and physicochemical mechanisms: (a) breakdown of cellular chloroplasts and chromoplasts, (b) changes in natural pigments (chlorophylls, carotenoids, and anthocyanins), and (c) development of enzymatic browning (Sinha et al., 2012).Fruit coloration is governed by several factors such as fruit maturity, tree nutrition, rootstock, cultivation practices, water availability, and temperature. Fruit color also depends on climatic conditions and groundcover in the orchard (Ladanyia, 2010). It is a unique phase in the life cycle of fruiting plants. it is one of the most important traits for both commercial and aesthetic value of fruit, mostly attributed to fl avonoids, betalains, carotenoids, and chlorophylls. Color development in fruits is an important evolutionary trait and a major factor contributing to fruit quality and subsequent market value. Coloration encompasses several physiological and biochemical changes that happen through diff erential expression of various developmentally regulated genes (Pomar et al., 2005; Torres et al., 2010).Major pigments that give color to citrus fruits are chlorophylls (green), carotenoids (yellow, orange, and deep orange), anthocyanins (blood red), and lycopenes (pink or red). During growth and maturation, especially in the immature stage, chlorophylls predominate in the peels of all citrus fruits. Due to the presence of chlorophyll, immature fruits are capable of photosynthesis, but they cannot make a signifi cant contribution to the own nutrition (Ladanyia, 2010).Animals and human cannot synthesize fl avonoids and carotenoids by themselves, so these health benefi cial substances must be obtained from their feeding on horticultural products, especially fruits. Modern technologies of genetic engineering or metabolic engineering may harness some microorganisms for industrial production of anthocyanins or carotenoids (Ajikumar et al., 2008), but it is uncertain those products will be accepted by consumers. Thus, it is reasonable to endeavor to develop the health-benefi ting colors of fruits. Flavonoids and betalains are water-soluble pigments, whereas carotenoids and chlorophylls are liposoluble.Citrus is among the most consumed fruits in the world and is rich in fl avonoids and carotenoids. To elevate the health-benefi cial values and the attractive appearance, new varieties of citrus accumulating fl avonoids and carotenoids in fruits have been brought to the world market continuously via breeding eff orts, such as selection from bud mutation, seedlings and nucellar lines, or sexual hybridization. In general, a large percentage of new varieties have been selected from spontaneous bud sports in the fi eld with improved fruit color caused by anthocyanin or carotenoids. Sexual hybridization programs have also been adopted to develop new varieties by taking at least one pigmented citrus as parent (Deng and Xu, 2011). The fl avonoids are an important class of plant secondary metabolites, belonging to the broader family of plant phenolics. No specifi c role can be assigned so far to the fl avonoids in the physiology of citrus. Citrus fl avonoids have aroused much interest due to their organoleptic properties (Spiegel and Goldschmidt, 1996).Over 5000 diff erent fl avonoids have been described to date and they are classifi ed into at least 10 chemical groups (Sinha et al., 2012). These are low-molecular-weight polyphenolic compounds that fl avones, mostly yellow fl avonols, fl avanones, fl avanols, isofl avones, colorless proanthcyanidins, and colorful anthocyanidins are particularly common in fruits (Le Marchand, 2002; Springob et al., 2003). Citrus fruits contain numerous fl avonoids with a typical pattern for each species, resulting good markers of the relative products and by-products. Most fl avonoids are in the fruit
728 Abouzar Abouzari, Nafi seh Mahdi Nezhadalbedo and fl avedo. And they can be grouped in fl avanones, fl avones, and anthocyanins (Horowitz and Gentili, 1977). The most numerous are the fl avanones which are, almost invariably, in the form of glycosides belonging to two groups: rutinosides and neohesperidosides (Tripoli et al., 2007). The fl avonoids may aff ect fruit quality characteristics such as texture, color, fl avor, and the nutritional value of fruits (e.g., in apples) through their involvement in the formation of undesirable brown pigments following bruising or cutting, due to the enzymatic oxidation of endogenous phenolics into quinone’s, which are then polymerized into brown products (Robards and Antolovich, 1997). Flavonoids increase the postharvest resistance of fruits to pathogens (Lattanzio, 2003), but may contribute to the formation of undesirable sediments in fruit juices and wine. Former investigation indicated that ‘Citrin’, a crude mixture of fl avonoids prepared from lemon peel, was eff ective in reducing capillary permeability in man, in prolonging life, and mitigating tissue hemorrhaging due to scurvy in guinea pigs. Among the many other clinical applications proposed for fl avonoids, it is worth noting its use, although of undemonstrated eff ectiveness, in the treatment of the common cold. The group of substances responsible for these eff ects was considered to be a vitamin, and was given the name ‘Vitamin P’. Successive research was unable to demonstrate that fl avonoids are necessary factors in the diet, and the term ‘Vitamin P’ fell into disuse, and was replaced by the term ‘biofl avonoids’ (fl avonoids having a biological activity). Flavonoids have been extensively studied for antioxidant, anticancer, antiviral, and anti-infl ammatory activities, eff ects on capillary fragility, and an observed inhibition of human platelet aggregation (Dugo and Di Giacomo, 2003).Citrus fl esh is the distinctive and edible tissue that contains anthocyanins, and these are sometimes visible as by the red blushes on the rind of blood orange. In variety development programs, more attention has been paid lately to fl esh coloration improvement caused by anthocyanins to improve fruit health quality. So far, only blood orange cultivars, such as ‘Budd’, ‘Moro’, ‘Tarocco’, ‘Sanguinello’, and so on, have been reported to produce fruits colored by anthocyanins (Lee, 2002; Rapisarda et al., 2009). Major anthocyanins in blood orange fruits and juice are cyanidin- 3-glucoside and cyanidin-3-(6-malonylglucoside). Additionally, minor anthocyanins and anthocyanin-derived pigments, such as 4-vinylcatechol adducts of cyanidin-3-glucoside, cyanidin-3-(3-malonylglucoside), delphinidin 3-glucoside, cyanidin 3-sophoroside, cyanidin -3-(6-dioxalylglucoside), have also been identifi ed (Hillebrand et al., 2004). Anthocyanins are the most prominent (Wheelwright and Janson, 1985) localized within the vacuole of the plant cell (Artes et al., 2002) largest and most diverse group of plant pigments including: cyanidin, delphinidin, malvidin, peonidin, and their various glycosides and acylated derivatives, and these provide a wide range of colors including violet, red, pink, blue, magenta, black and that derived from the phenyl propanoid pathway. Anthocyanins are always produced during fruit ripening, but in some fruits such as nectarines (Prunus persica [L.] Batsch.), they are produced during the fi rst stages of fruit development. The anthocyanin content of ripe fruit is aff ected by environmental conditions such as light and temperature (Faragher, 1983; Arakawa et al., 1985). Most studies reported on anthocyanin biosynthesis mechanism are in apple and grape (Matus et al., 2008), and there is no clear study reported on other fruits. It’s probably due to some genes involved in anthocyanin biosynthesis having pleiotropy and being aff ected by many internal and external factors. Transgenic approaches have discovered a strong relationship between phenyl propanoid/fl avonoid gene expressions for fruit skin coloration (Kayesh et al., 2013). Investigation in grape indicated that higher phenol and anthocyanin content was directly correlated with higher antioxidant activities (Alcolea et al., 2002). Anthocyanins are one class of fl avonoid compounds that act as powerful antioxidants so are able to prevent oxidative damage to proteins, lipids, DNA, and other macromolecules caused by reactive oxygen species (ROS). Thus besides the classical use of inhibitors or the isolation of mutants for scavenge the toxic ROS, manipulation of the antioxidant enzyme levels in specifi c cell by genetic tools can infl uence the accumulation of ROS in given cellular compartments. Maintenance of a high antioxidant capacity to scavenge the toxic ROS has been linked to increased tolerance of plants to environmental stresses (Abouzari and Fakheri, 2015). Several methods are used to measure antioxidant activity. The most commonly used are those that use chromogen radical compounds to simulate reactive oxygen and nitrogen species. Anthocyanins are easily lost by lixiviation when the cell membranes break down. Also oxidation can play an important role in anthocyanin degradation catalyzed by light (Sinha et al., 2012).Carotenoids are lipid-soluble plant pigments, common in photosynthetic plants. The term carotenoid summarizes a class of structurally related pigments, mainly found in plants. At present, more than 600 diff erent carotenoids have been identifi ed although only about two dozen are regularly consumed by humans. The most prominent member of this group is -carotene. Normally, carotenoids as effi cient photoprotective agents, quench the excited state of chlorophyll, thus preventing the formation of singlet oxygen. Little is known about carotenoids in ROS detoxifi cation in plants. However, overexpression of ß-carotene hydroxylase in Arabidopsis leads to increased amounts of xanthophyll in chloroplasts and results in enhanced tolerance towards oxidative stress induced in high light (Abouzari and Fakheri, 2015). The carotenoids of citrus fruits have been extensively
The Investigation of Citrus Fruit Quality. Popular Characteristic and Breeding 729investigated. The Citrus genus is remarkable for producing the largest number of carotenoids found in fruit. About 115 diff erent pigments have been reported, including a large number of isomers, some of which might be formed during isolation. Each species and hybrid has a characteristic carotenoid complex which is responsible for its typical color; peel and pulp pigments reveal certain diff erences. In yellow citrus fruits (pummelo, grapefruit, lemon, lime), the total amount of carotenoids is low and most of these belong to the colorless carotenoids. Thus, in white Marsh seedless grapefruit the colorless phytoene and phytofl uene account for 74% of the carotenoids of the peel. Orange-colored citrus fruits (orange, sour orange, mandarin) contain relatively large amounts of a complex mixture of carotenoids (Spiegel-Roy and Goldschmidt, 1996). There is a rapid synthesis of carotenoids in the chromoplast during ripening, which is accompanied by a simultaneous loss of chlorophyll. With ripening, total carotenoids increase in the peel as well as in the pulp. Rind of fruit is the region of higher carotenoid concentration, and 50–75 percent of the total carotenoids of oranges exists in the peel. (Ladanyia, 2010). Carotenoids are aff ected by pH, enzymatic activity, light, and oxidation associated with the conjugated double bond system. The chemical changes occurring in carotenoids during processing have been reviewed by several authors (Simpson, 1986; Rodriguez-Amaya, 1997).In citrus, natural mutants with red peel and juice vesicles have been found mainly in Texas grapefruit. Mutation breeding using thermal neutrons has brought about a most signifi cant change from a deeply coloured, highly seeded grapefruit to a seedless one. In another, more recent case, thermal neutrons and natural mutation have been instrumental in producing a mutant with an increased red colour from an established natural mutant with a good peel and fl esh colour. Of the other fruit characteristics, mutants for low acidity are of considerable interest in citrus, and particularly in grapefruit (Jackson, 1987). In the apple, low acid types depend on a single homozygous recessive gene (Brown, 1975).The world grapefruit market has shi ed signifi cantly toward seedless high-pigmented colored types. The US and EU market almost exclusively requires cultivars with a red pulp high in carotenoids. The Japanese market is another traditionally strong grapefruit market that is shi ing to pigmented grapefruit although the non-pigmented types still have a high demand. Other quality parameters important in international marketing of citrus fruit include peel fi rmness and juice content in lemons and limes (Picha, 2006). Genes involved in metabolic pathway regulation, such as those in the fl avonoid pathway, have also been introduced in citrus (Costa et al., 2002). The growing interest in manipulating carotenoid biosynthesis in plants is mainly related to human nutrition as precursors of vitamin A and natural antioxidants. Also, this kind of pathway manipulation holds promise of altering color and fl avor development in citrus.SeedlessnessIn some plants seedlessness is attributed to abortion of embryos produced by fertilization, a phenomenon known as stenospermocarpy, which requires both normal pollination and fertilization. Seedlessness can also result from parthenocarpy, wherein fruits set without fertilization. On the other hand, parthenocarpic fruit development may or may not require pollination, but most seedless citrus fruits require stimulation from pollination to produce fruit (Jenks and Bebeli, 2011). Certain environmental factors, i.e., low or high temperatures, chromosomal aberrations, chemical treatment, and some genetic factors, like gene(s) controlling meiosis process can also induce seedlessness (Lin et al., 1984; Nuez et al., 1986). On the other hand, self-incompatibility may be combined with trait(s) of parthenocarpy. High endogenous auxins levels and GAs arise in natural parthenocarpic citrus cultivars (George et al., 1984; Talon e t al., 1992).Among the diff erent criteria of citrus quality, seedlessness is a major characteristic and is a highly desirable trait for fresh fruits. There are many desirable characteristics of seedless fruit, which include high quality and taste, greatly valued by both consumers and the processing industry. Commercial cultivars of bananas (Musa acuminata) and pineapples (Ananas comosus) are examples of seedless fruits. Seedless cultivars of citrus, grapes (Vitis vinifera), and watermelons (Citrulus lanatus) are highly valued by consumers. The presence of a large number of seeds in citrus fruits greatly hinders consumer acceptability, even if it has good organoleptic properties. Therefore, the development of seedless fruit cultivars has become a major goal for fruit breeders around the world and diff erent approaches have been adopted by numerous researchers. Genes that induce seedlessness in fruit are being major objective in some quest (Koltunow et al., 2000). Considerable breeding work has been done to develop new seedless cultivars. These consist of both orthodox and modern molecular breeding tools. Conventional breeding in citrus has important limitations due to the complex reproductive biology of these species and was also time exhaustive. Most genotypes are apomictic, and adventitious embryos develop directly from nucellar cells limiting or precluding the development of zygotic embryos. This limits the recovery of large sexual populations, and in practice, apomictic genotypes are avoided as female parents in many programs.Several high-quality genotypes have pollen and/or ovule sterility and thus cannot be used as parents in breeding programs. Self- and cross-incompatibility are relatively common among many genotypes, also limiting the possibilities to select parents for specifi c crosses. Citrus has a long juvenile phase and in most species at least fi ve years
730 Abouzar Abouzari, Nafi seh Mahdi Nezhadare required to start fl owering and many more to completely lose the undesirable characters, such as thorns, associated with juvenility. These biological factors and the diffi culty to manage fi eld evaluation for large progenies and multigenerational breeding schemes are the main reason for the relatively low success of conventional breeding programs. The seedlessness of citrus varies among accessions and sometimes environmental conditions. ‘Navel’ orange and ‘Satsuma’ mandarins are usually seedless, but occasionally they produce seeds when pollinated. Information regarding genetic and molecular aspects aff ecting the gamete fertility and seed development is now increasing rapidly and will defi nitely enhance the potential of breeding for seedlessness through sexual crosses at diploid level. Seedlessness in citrus mandarin cultivars can be induced by many factors, including female or male sterility, defective ovules and embryo-sac abortion, pollen self incompatibility, polyploidy, abnormal climate and application of plant-growth regulators, such as gibberellins. Many seedless citrus cultivars, especially seedless mandarin cultivars, originate from the bud mutation of seedy cultivars (Shen, 1997).Easy of PeelingConsumer appear to be less willing to spend time preparing fruit for consumption (i.e. peeling, slicing, removing seeds, etc.), but are willing to spend more for ready -to-eat (i.e.”fresh-cut’’).In citrus breeding, in addition to color and seedlessness, easy of peeling is another essential traits and selection objectives for fresh market cultivars (Stover et al., 2005).The combined form of albedo (mesocarp) and fl avedo (epicarp) is called the pericarp, commonly known as the rind or peel. From the molecular point of view, pectin, cellulose, and hemicellulose are responsible for the adherence of the skin to the fruit (Whitaker, 1984). In some varieties of orange and mandarins, the albedo is very thin and diffi cult to separate from the fl avedo, a fact aff ecting the enzymatic peeling. Enzymatic peeling is an alternative method for removing the rind of the fruits with lower water consumption and causing less contamination than the traditional methods. The presence of the navel hampers the diff usion of the enzymatic solution into the tissue and the irregularity of the segments decreases the quality of the fi nal product, as could be verifi ed in the Thomson variety (Pretel et al., 2007).Mandarins are an easily peeled citrus fruit due to the weak adherence between epicarp and endocarp. These fruits are widely cultivated in the world, especially for their fresh consumption although some varieties like Satsuma are commercialized advertised in syrup (Bayindirli, 2010).A fairly large group of mandarin hybrids has been found and propagated, and also produced by breeding. These are treated in commerce in a manner similar to mandarin or ‘easy peeling fruit’. Those originating from a cross between grapefruit and mandarin are called ‘tangelos’. Grapefruits and Valencia oranges can be stored for three to fi ve months and green lemons even longer, but many easy peeling, mandarin-like cultivars cannot be stored for longer than a few weeks. so long time of stored must be considered in breeding program for easy peeling (Spiegel-Roy and Goldschmidt, 1996).Enzymatic peeling is an alternative method for removing the rind of the fruits with lower water consumption and causing less contamination than the traditional methods. The principle of the enzymatic peeling is based on the digestion, by an enzymatic preparation, of substances that found in cell walls of plants and responsible for the adherence of the skin to the fruit. Therefore, both pectinases and cellulases are needed for the enzymatic peeling. The cellulases are probably needed for the release of the pectins in the albedo, and the pectinases contribute to the hydrolysis of the polysaccharides of the cell wall.Fruit SizeSize is of foremost economic signifi cance for marketing of fresh fruit, particularly with small, easy-peeling cultivars like ‘Clementine’. Fruit of the genus Citrus vary greatly in size and range from about 30 mm in diameter for kumquats (Fortunella spp.) to more than 300 mm in diameter for pummelos (C. grandis) (Ray, 2002). Fruit size in mandarins can vary from small to large, but most fruit have a size of between 50 mm and 80 mm in diameter.Seed content also plays a role in fruit size. A linear relationship between fruit size and seed number is common in citrus which indicates a strong infl uence of seeds on fruit development. Consequently, fruit size is o en sacrifi ced when seedless fruits are desired (Vithanage, 1991).Seedless fruit are generally small and an increase in the seed content can increase the fruit size. Since fruit development is linked to the development of the ovule, it is possible that a hormonal stimulus from the seeds regulates fruit growth. Pollen has been found to play a role in fruit size and generally larger fruit with more seeds are produced if more pollen is applied to the stigma of the fl ower. Almost every possible agrotechnique has been tested in an attempt to increase fruit size. A large amount of photosynthate is required for fruit enlargement. Larger fruit are, therefore, found on leafy, compared to leafl ess, and branches due to the nearby leaves being potent suppliers of photosynthate (Ladaniya, 2010). Mechanical practices such as girdling and fruit thinning can be used to increase the amount of photosynthate available to the fruit, and therefore, increase fruit size. Girdling involves removing a ring of bark from the trunk or scaff old branches interfering with the downward phloem transport and preventing the escape of the photosynthate from the girdled area to other parts of the tree. Girdling during the fruit enlargement stage can increase the
The Investigation of Citrus Fruit Quality. Popular Characteristic and Breeding 731fruit size by up to 30%. Fruit thinning, where some of the fruit are removed, increases the leafy area per fruit making more photosynthate available to each individual fruit and thereby increasing the fruit size. A several-fold increase in fruit size may be obtained when girdling is combined with extreme fruit thinning.Plant growth regulators have proved eff ective for increasing fruit set, checking fruit drop, thinning out, keeping the fruit on the tree for longer periods, increasing the postharvest life, and infl uencing fruit quality. The eff ectiveness of these plant growth regulators, however, is infl uenced by the climatic conditions and the citrus varieties to which they are applied. Plant growth regulators for example auxins can be used to manipulate fruit size. Fruit size increased with auxins in three ways: fi rstly by thinning the crop and increasing the growth of the remaining fruitlets; secondly when applied at the end of the fi rst stage and beginning of the second stage of fruit development, they directly enhance fruit growth; and thirdly when applied to the stylar end of young fruit they enhance the growth of some fruitlets and increase late fruitlet abscission (Ladaniya, 2008).Mineral nutrition is another factor infl uencing fruit growth and the macronutrient potassium plays an important role in fruit size. A potassium defi ciency in the tree results in smaller fruit, and potassium sprays are used commercially to increase the fruit size. Several horticultural practices such as girdling the stems, spraying with plant growth regulators, and hand thinning of fruit are used to reduce the eff ect of this irregular bearing behavior (Saunt, 2000).Intrinsic fruit size is probably a genetic trait while the fruit number refl ects the tree’s bearing limits. Even though the yield varies considerably between citrus cultivars, all cultivars have shown the fruit size to be inversely proportional to the number of fruit on the tree (Spiegel-Roy and Goldschmidt, 1996), with heavy bearing trees producing smaller fruit (Ladaniya, 2008).In oranges, an inverse relation between size, soluble solids, acidity, and ascorbic acid content has been established. No appreciable diff erence in the contents of sugars, ascorbic acid, or naringin was found in the large and small fruits of Japanese Natsudaidai, but the small fruits were higher in total and amino nitrogen. It was reported that large and medium sized Satsuma mandarins contained higher percentage of soluble solids and titratable acid, and had a better color than the small mandarins (Ramana et al., 2009).Major Breeding StrategiesThe basic features of any eff ective quality assessment in a plant breeding program is that they should be quick, cheap, and use very little material. These three criteria are important because (Brown and Caligari, 2011): 1) A plant breeding program will involve screening many thousands of breeding lines each year or growing cycle. 2) A plant breeder is o en working against time. Many quality traits are assessed post-harvest and it is o en important to make selection decisions quickly, before large-scale quality is determined. The development of new and improved citrus cultivars by conventional methods is a slow and costly process. It may take as long as 20–35 years or longer to release a new cultivar from the time of making the cross (Khan, 2007). 3) In most stages of a plant breeding scheme, there are only limited amounts of material available for testing. It is o en the propagative parts of the plants (seeds or tubers) that are used for testing, and so a further complication is that many of the quality tests available are destructive of the very parts of the plant that are required to be grown to provide the next generation for selection. Despite the fact that citrus breeding is very challenging, diff erent breeding programs throughout the world have made signifi cant progress in the application of conventional and modern approaches to genetic improvement and cultivar development. Important breeding goals exist in citrus with respect to both scions and rootstocks. Citrus breeding methods include bud and seedling mutation selection, mutagenesis, hybridization, and various biotechnologies (Deng and Xu, 2011).Conventional methods of breeding scion and rootstock cultivars are generally based on controlled crosses. To combine desirable traits from diff erent selections, cultivars, or species in hybrid progeny, cross-pollination is carried out. After the hybrid fruits have matured, the seeds are extracted from the fruit and planted in the greenhouse. Once the seedlings have attained sufficient size, they are either graded onto rootstocks or directly planted in the field for evaluation of their performance. The hybrids first are evaluated for some important treats includes disease and pest resistance, stress tolerance, and overall growth characteristics during the juvenile period, then subsequently for fruit characteristics of scions or rootstock traits of interest.
Clonal Selection.
Clonal selection can be used to isolate superior bud source strains of established cultivars and to eliminate propagation of undesirable budlines. Clonal selection within cultivar groups (‘budline selection’) has been useful for the development of improved strains of cultivar groups like Satsuma mandarin and navel sweet orange (Jain and Pryadarshan, 2009).Use of New Self-incompatible Cultivars Phenomenon of self-incompatibility is genetically controlled which prevents seed set in self-pollinated crops and produce functional gametes. Usually in condition of self-pollination, no properly developed pollen tubes were observed in the ovaries of self-incompatible plants (Ton and Krezdorn, 1967). The incompatibility system of citrus is of gametophytic nature and incompatibility ‘S’ allele(s) are widely distributed; not only in self-incompatible cultivars but also in self-compatibles like satsuma mandarins and ‘Dancy’ grapefruit (Soost, 1968; Vardi et al., 2000). Self-incompatible accessions have the ability to produce seedless fruits when cross pollination is prevented. However, those accessions may produce seedy fruits in mixed plantings particularly in present of pollinating insects. There is little information available on the inheritance of self-incompatibility in citrus, however, it has been found that hybrids between self-incompatible cultivars have also been self-incompatible and sometimes cross-incompatible (Soost and Cameron, 1975). Sterility can be classified into three types (Yamamoto et al., 1995): self-incompatibility, female sterility, and male sterility. Gametophytic self-incompatibility is common in pummelo and is found in several mandarin and mandarin hybrid cultivars (Soost, 1968). Male and female sterility results in the complete inability to reproduce by means of seed and may be due to different genetic factors such as triploidy, sterility genes, and chromosomal abnormalities such as reciprocal translocations and inversions. Some male-sterile and self-incompatible accessions cannot produce seedless fruits because of the lack of parthenocarpy. Thus, parthenocarpy is an indispensable trait for seedless fruit production, and this character seems to be widely present in citrus germplasm. Autonomic parthenocarpy, where seedless fruit is produced without any external stimulation (pollination), is the main type of parthenocarpy in citrus (‘Navel’ orange or ‘Satsuma’ mandarin), but stimulative parthenocarpy has also been reported (Vardi et al., 1988). The importance of self-compatibility for achieving better crops, at least in certain seasons and environments, and for better orchard management (dispensing with lower value pollenizers), is well recognized. ‘Clementine’, ‘Fortune’ mandarin, and ‘Afourer’ tangor are good examples of such self-incompatible cultivars. However the list of self-incompatible cultivars is on the increase, and with the ancestry of many cultivars unknown, the presence of incompatibility in many progenies cannot be predicted. The main default of these cultivars is their ability to be cross-pollinated and to produce seedy fruits when they are cultivated in multicultivar orchards (Janick, 2008). Among the citrus species and cultivars, the percentage of functional pollen is very diff erent. Lemon and other orange cultivars have low amounts, Marsh grapefruit have very little pollen, while mandarin and pummelo produce mostly functional pollen. Satsuma mandarins and navel oranges are mostly pollen sterile and set parthenocarpic fruit. Cultivars with non-functional pollen usually show ovule abortion although Washington navel and satsumas have functional ovules. Pollen degeneration before meiosis is also encountered (Spiegel-Roy and Goldschmidt, 1996; Ray, 2002).Use of Triploid Varieties. The first chromosome studies of the genus Citrus were reportedly conducted by Strasburger in 1907 who recorded the haploid number as eight. Diploidy with somatic chromosome number 2n = 18 is the norm for Citrus and closely related genera. There are a high degree of morphological variability between species, and between varieties within the species. Various Citrus euploids have been reported. Monoploid plants of several Citrus species and of Poncirus trifoliata have been derived from pollen culture in vitro. Monoploid seedlings also arose after gamma irradiation of seeds. Triploids is one of the most common polyploids of citrus, discussed in this paper (Lee, 1988). Triploids have generally been considered to be an evolutionary dead end because they have very low fertility and tend to produce aneuploid gametes, due to problems of chromosome pairing during meiosis. Citrus species are consistently diploid, but a low frequency of triploid and tetraploid seedlings occur in their progenies. Triploids are particularly important because they are seedless. In the case of citrus, early cytogenetic studies have described triploid meiosis. Trivalent pairing and some univalents were observed and described a pre dominance of trivalents but also the presence of numerous bivalents and univalents as well as a great variation of the number of extra microspores in some genotypes. The selection of triploid lines has been, and is already, a very interesting way to develop seedless cultivars (Ollitrault et al., 2008). Triploid vigour is refl ected in the production of large fruits and, in the case of the triploid citrus genotypes, in seedlessnes (Cannell and Jackson, 1985). Indeed triploidy is generally associated with both male and female sterility. Thus, most of the trees under field evaluation present these characteristics, and an efficient selection can be carried out in other traits. Moreover larger fruit size associated with triploidy should correct the reduction of fruit size generally observed in seedless mutants of seedy cultivars. Triploids can produce haploid, diploid, or triploid gametes at low rates, which can lead to diploid, triploid, and tetraploid progenies (Otto and Whitton, 2000).Several methods have been developed for triploid citrus creation: One of them exploits natural events of polyploidization such as 2n gametes, while the more recent strategies combine haplomethods such as androgenesis or induced gynogenesis, somatic hybridization for a direct synthesis of triploid hybrids, using embryo rescue and flow cytometry to select triploids in 2x × 2x crosses (Ollitrault et al., 2008).Second meiotic division restitution has been proposed for diploid megagametophyte development in clementine (Luro et al., 2004) while Chen et al. (2008) reported first meiotic division restitution in sweet oranges. The classic strategy is to cross diploid monoembryonic females with tetraploid males. Such tetraploid plants can be found in apomictic seedlings (natural doubling of the chromosome stock of nucellar cells) or are created by somatic hybridization (Grosser et al., 2000). In the past this strategy was limited by the scarcity of tetraploid genitors. Today however, the pool of tetraploid genitors can be substantially enriched with new, highly heterozygous tetraploid genotypes obtained by somatic hybridization (Tusa et al., 1996).Another possible source is endosperm in vitro culture, which has also enabled the recovery of triploid plants. However, this technique seems poorly adapted for large scale breeding programs (Ollitrault et al., 2008). Recently, tetraploid monoembryonic lines have been obtained by in vitro colchicine treatment of shoot tips gra ed in vitro (Juárez et al., 2004; Navarro and Juárez, 2007). While Wakana et al. (2005) obtained tetraploid branches by top-grading shoots with sprouting axial buds treated with colchicine. In this way these authors avoid the juvenility of seedlings and have been able to use these tetraploids as parents two to four years after colchicine treatments. It appears that 4x × 2x crosses have been more successful in producing triploid embryos (Cameron and Frost, 1968; Soost and Cameron, 1975). It was proposed that the 5:3 ratio between endosperm and embryo ploidy obtained with these last crosses was more favorable than the 4:3 obtained in the reciprocal ones. These tetraploids open the avenue to 4x × 2x crosses with the maternal tetraploid Clementine. Tetraploid plants have also been regenerated by somaclonal variation from C. limon embryogenic callus obtained from ovules culture on medium containing 2, 4-D (Vardi and Spiegel-Roy, 1982). In the last 10 years, several new triploid cultivars resulting mostly from diploid × autotetraploid sexual crosses have been released in Italy, the USA, and Japan (Badenes and Byrne, 2012). The current ploidy manipulation programmes will result in an extensive range of triploids (seedless) citrus cultivars and will be utilized to combine trait of seedlessness and other characters, i.e., resistances against insect pest and diseases, quality traits or extended harvesting period. French Agricultural Research Centre for International Development (CIRAD) has used somatic hybridisation between diploid and haploid lines. This method makes it possible to add, without recombination, a haploid genome to the whole diploid genome of high organoleptic quality cultivars. Somatic hybridisation is done by electrofusion of protoplasts isolated from haploid embryogenic callus lines of clementine, obtained from haploid embryos generated by induced gynogenesis, and callus lines or mesophyll protoplasts of diploid cultivars (Ollitrault et al., 2008).Mutation breeding by irradiation is also a suitable approach to pursue desired triploids because natural mutations and bud sprouts has o en observed in citrus. Gamma irradiation was employed to obtain somatic mutations to study the evolution patterns of several citrus varieties and species. Although polyploidy o en occurs naturally in citrus through spontaneous mutations, the condition of triploidy has occurred only one case of cultivated citrus (‘Tahiti’ Lime) (Recupero et al., 2005).Use of Mutation. Mutation induction has arisen as a useful technique to increase genetic variability in plant populations, not only in citrus, but also in other cultivars of commercial relevance. The International Atomic Energy Agency has registered more than 2000 different mutant cultivars officially released and obtained through irradiation mutagenic programs. Several studies have shown that irradiation induced a large number of functional variations in plants although the efficiency depends on the dosage, type of mutagen and species (Iglesias et al., 2009). Mutation was conceived as a complementary method to conventional breeding. Spontaneous mutations have been reported in various citrus fruits (e.g. oranges, lemons, mandarins). Selection of spontaneous mutations is the oldest citrus breeding method and most of the varieties cultivated worldwide arose from this process. For the induction of mutational events in plant material the mutation breeder can choose between two groups of mutagenic agents, namely, chemical and physical mutagens. The latter have been used for many decades, whereas the use of chemicals is relatively recent, starting in approximately 1940 (Broertjes, 2012). Radiation is the most widely used type of mutagen for citrus. The two most commonly used radiation types are X-rays and -rays (generally from a 60Co source). Dose is the amount of mutagen applied per unit time while exposure is the total absorbed radiation. Radiation has been found to induce many non-lethal chromosome aberrations that often affect more than one gene. Gamma irradiation has been the most common method of mutagenesis. It can induce a wide range of mutations (point mutation, chromosomes breaks, and rearrangements). The latter type is particularly interesting for seedless selection (Hearn, 1984) because translocations and inversions may cause sterility. Roose and Williams (2007) recommend exposure of budwood to 30–50 Gy to induce seedless mutations. Classical examples of cultivars obtained by irradiation programs are the Star Ruby red flesh grapefruit obtained by irradiation of seeds of Hudson grapefruit (Hensz, 1971), and more recently the low-seeded selection of Murcott, called ‘Mor’ mandarin produced by budwood mutation (Vardi et al., 1993), and the seedless mandarin Tango produced by irradiation of Afourer (Nadorcott) budwood (Roose and Williams, 2007).Chemical mutagens have been used less commonly in citrus than radiation (Broertjes, 2012). Different chemical mutagens induce different spectra of mutations. A very wide range of chemical are now available, but few of these have been evaluated in citrus. The breeder can initiate a mutation breeding project with one of several different types of tissues, each with certain advantages and disadvantages (Tab. I). Choice of tissue may also depend on the target trait and type of mutagen to be used. Pollen irradiation has rarely been used in citrus. In versus treatment of buds has been widely used in citrus (Froneman et al., 1996). A major advantage is that trees propagated from such buds will not display the delayed flowering and thorniness typical of seedlings. However, the probability of chimerism is high relative to treatment of single-cell systems (Khan, 2007). Mutant genotypes may be obtained that are improved in one trait and poorer in other characteristics. This imposes the need for large vegetative progenies, and the selection and observation of a larger number of mutants (Donini, 1982).Nearly all work on inducing seedlessness through mutation breeding has been performed in citrus. The most important natural seedless mutants have been found in various citrus species (Spiegel-Roy et al., 1985; Spiegel-Roy, 1990). Induced mutagenesis of seedy cultivars have been developed in several countries and gave good results for seedless line selection (Chen et al., 1991; Froneman et al., 1996; Uzun et al., 2008). Seedlessness is usually, but not invariably, associated with high pollen and ovule sterility. However, the quality, economic value and marketability in citrus will increase with a decrease in the number of seeds per fruit. In citrus, a pronounced parthenocarpic tendency is essential for satisfactory fruit set and yield. Problems of parthenocarpic fruit set and the size of the yield are o en linked to seediness, at least with certain genotypes. Generally the seedless mutants should produce fruits significantly smaller than the parental seedy cultivar. These effects on the decrease in fruit size are in numerous cases not severe enough to detract from economic importance. The points to the need for making a larger number of selections from the same genotype when selection is performed for seedlessness. The time (years) required for final selection and assessment of the economic value will also have to be increased. Because the seedlings will be juvenile, it may be difficult to distinguish mutants from recombinants in the sexual progeny that result. Induction of seedlessness has been achieved by treating apomictic seeds with gamma rays (Hearn, 1984), seeds with thermal neutrons (Hensz, 1977), and scions with gamma rays (Hearn, 1986; Spiegel-Roy et al., 1985). Somatic mutations have played an important role in the development of new cultivars, partly because apomixis is common in most Citrus species through the formation of nucellar embryos which derive from somatic cells of the nucellus. Seedlings may be of zygotic or non-zygotic (nucellar) origin. The percentage of seedlings that are of non-zygotic origin varies greatly, o en being high to very high and very rarely zero (Soost and Cameron, 1975).Mutation breeding by irradiation of seeds and/or axillary buds has also given rise to seedless clones of normally seedy ‘Pineapple’ orange, ‘Duncan’, and ‘Foster’ grapefruit, ‘Monreal’ Clementine mandarin, ‘Eureka’ lemon, and ‘Kutdiken’ lemon. A seedless mandarin ‘Tango’ has been produced by irradiation of buds of W. Murcott. ‘Monreal verde’ is an almost seedless variety obtained by irradiating budwood of ‘Monreal’ Clementine (Jain and Pryadarshan, 2009).Natural mutants of improved color are of great significance in orange and grapefruit. Nucellar selection accompanied by thermal neutrons enabled the development of two mutants of great commercial significance and prospect. Star Ruby exceeds all natural and induced mutants in colour, it has been derived from a highly seeded cultivar (Hudson Red), with an intense internal and external colour. Rio Red, also seedless, has been derived from Ruby Red, a coloured, seedless mutant, and is now being ranked by most experts as second only to Star Ruby in color, with better possibilities of adaptation to the environment and cultural practices (Novak, 1991).Scion Breeding. The scion breeding programs are mainly aimed at improving the fruit quality include: flavor, size, color, shape, and yield, as well as low seed content, easy peeling, and disease resistance. The main breeding aims for scion cultivars vary with species and localities. The first step in scion breeding involves selection of parental types with favorable heritable characteristics/traits. O en those seed parents are selected that produce only zygotic progeny (Soost and Cameron, 1975). Hence monoembryonic parents are preferred for scion breeding. Mandarins are relatively easy to breed by crossing parents with good traits and selecting superior progeny. The seeds from mature hybrid fruits after extraction, separately planted in greenhouse carefully and after seedlings have I: Advantages and disadvantages of treating various tissue types with mutagens (Khan, 2007)Target tissue Ease of use Time to evaluation Chimerism Identification of mutants Pollen Good Long None Difficult Seed Good Long Low Varies Embryogenic callus culture Fair Long Low Easy Mature stem segment culture Fair Short High EasyBudwood Good Short High Easy attained sufficient size, they are then planted in the field. Once the desired scion hybrid is selected, it is budded onto different rootstocks to further evaluate its performance. The hybrids are also tested for biotic and abiotic stress tolerance, and their overall growth characteristics are also monitored. Sexual hybridization will continue to receive attention for mandarin cultivar development because of increased numbers of improved monoembryonic breeding parents available for hybridization. Interspecific hybrids between mandarin-sweet orange (tangors) and mandarin-grapefruit (tangelos) have been developed by breeding programs (Gmitter et al., 1992).Many of the widely grown scion cultivar groups, such as sweet orange, grapefruit, lemon, and various clonal selections of certain mandarin cultivars such as ‘Satsuma’ and ‘Clementine’, originated as either bud sport mutations or apomictic seedling mutants. No cultivars of these have ever originated as sexually derived seedlings (Gmitter et al., 1992).Rootstocks BreedingBeyond productivity, the rootstock choice has a strong impact on fruit quality. It can affect the sugar and acid content, the fruit size, and the percentage of juice content. For example Poncirus and its hybrids are known to favor high quality of mandarin and orange production, while C. macrophylla is very productive but reduces fruit quality. Thus productivity and fruit quality of the associated scions are an essential criteria for rootstock selection. However, choice of rootstock is not usually based on fruit quality considerations alone; disease tolerance, soil type, and effects on yield are more often overriding considerations. The major objectives of rootstock breeding are aimed to control the tree size and to improve resistance and tolerance to biotic and abiotic stresses. Like scion breeding, rootstock breeding also involves controlled crossing. However, in rootstock breeding, highly polyembryonic species are selected as parents or at least one parent which is polyembryonic. Potential rootstocks showing favorable qualities are planted (Jain and Pryadarshan, 2009).New Breeding Techniques Conventional cross breeding can be considered foundation of citrus variety improvement. However, this method cannot be used to develop improved cultivars in many economically important citrus species such as sweet orange, grapefruit, and lemon due to barriers of sterility, self-and cross-incompatibility, and the widespread polyembryony. In addition to this, the heterozygosity makes the breeding for specific traits extremely difficult. Traditional breeding approaches via sexual hybridization have not been useful for the genetic improvement of most of the citrus cultivars. Therefore citrus variety improvement programmes have in the past relied on limited sources of genetic variation. In addition to conventional breeding they have included spontaneous mutations, irradiation of seed and budwood and importing germ plasm from other locations. Nowadays new directions have been opened by biotechnology. Modern biotechnologies based on in vitro cell, protoplast, tissue, and organ culture, and gene introduction, are being tried to improve the quality of existing cultivars and to produce new varieties. The modern tools of molecular biology should throw more light on the functions of enzymes, their pathways, and the genes controlling them. Using genetic engineering techniques, metabolic pathways can be
modified with certain enzymes, forming desired metabolites and eliminating problems related to the seedless citrus cultivars, flavor and eating quality of fruits (Ollitrault et al., 2008). It is a pleasant surprise that in recent years several genetic, genomic, and proteomic tools and technologies have been quickly adapted by the citrus research community to address major challenges of this plant system. Micropropagation Application of the biotechnological sciences such as regeneration and micropropagation, somaclonal variation, somatic hybridization, ploidy manipulation, transformation, genomics has the potential to unlock an entirely new round of genetic improvements for citrus crops.The responsibility of citrus to be regenerated via organogenesis and somatic embryogenesis is the fundamental basis that makes possible much of the potential for advances in plant cell and tissue culture. Somatic Embryogenesis Somatic embryogenesis is particularly attractive in citrus because many genotypes have the capacity for nucellar embryony (Soost and Roose, 1996). Somatic embryogenesis has been induced directly in cultured nucelli and undeveloped ovules or indirectly via callus formation. Embryogenesis has also been induced from endosperm-derived callus, juice vesicles, styles, anthers and pistil thin cell layers (Jain and Pryadarshan, 2009).The identification of valuable somaclonal variants holds great promise for cultivar improvement especially for the citrus species that are difficult to manipulate by sexual hybridization (Gmitter et al., 1992). Somaclonal variation has been observed in citrus plants regenerated from nucellar callus of monoembryonic ‘Clementine’ mandarin (Jain and Pryadarshan, 2009).
Somatic Hybridization.
Somatic hybridization allows production of somatic hybrids that incorporate genomes of the two parents without recombination, thus avoiding the problem of the high heterozygosity in citrus. In citrus, this technology has been extensively used and has many important implications. It has made production of hybrids from sexual incompatible or difficult to hybridize citrus relatives that possess valuable attributes possible, thus broadening the germplasm base available for rootstock improvement. Somatic hybridization has also been used to create new tetraploid somatic hybrids that combine elite diploid scion material to be used as tetraploid breeding parents in interploid hybridization schemes to develop seedless and easy-to-peel new mandarin varieties. Another approach to seedlessness is the transfer of cytoplasmic male sterility from Satsuma oranges to other elite but seedy scions via cybridization. This approach has the potential to make existing popular cultivars seedless, without altering the cultivar integrity in any other way. Somatic hybridization is now involved in five primary strategies to develop improved citrus varieties. For scion improvement, the primary strategy is to produce allotetraploid breeding parents by combining complementary elite scion varieties. Pollen from such hybrids can be used in interploid crosses with selected monoembryonic diploid females to produce seedless triploid hybrids for selection. A second way for producing improved scions is to produce triploids directly by haploid + diploid protoplast fusion. Two strategies being employed for rootstock improvement are: 1) to produce somatic hybrids of complementary rootstock parents that have potential for improved disease resistance, tree size control, and horticultural performance and 2) to produce wide hybrids of citrus with related genera to broaden the germplasm base, including sexually incompatible or difficult to hybridize citrus relatives. A final option is the production of citrus somatic cybrids, which may have potential in both scion and rootstock improvement (Grosser et al., 2000).Transformation and GenomicsGenetic transformation may provide an efficient alternative for citrus improvement, opening the way for the introduction of specific traits into known genotypes without altering their elite genetic background. The systems of transformation that have been used for genetic engineering experiments are dependent upon the fundamental abilities of in vitro regeneration. Genetic engineering has been applied to an increasing number of traits for citrus improvement (Jain and Pryadarshan, 2009).The entire field of citrus biology and genetics can be revolutionized by expanding the potential capabilities of genomics and bioinformatics to cultivar improvement through precise and targeted manipulations of the genome. The rapid development of molecular marker technologies has made it possible to investigate gene expression, and has helped in construction and integration of genetic and physical maps of the economically important traits such as CTV resistance (Gmitter et al., 1996). Molecular marker technologies also provide tools to tag the genes of known phenotypes by developing localized molecular linkage maps. These are very essential for map-based cloning (MBC) approach and marker-assisted selection (MAS) breeding programmes (Asins, 2002). MBC is also called as positional cloning. It is another approach to isolate gene(s), without prior knowledge of gene product, using tools of comprehensive genetics, genomics, and bioinformatics. Molecular markers have also been widely applied on phylogenetic and taxonomic studies in citrus. Integration of genetic linkage maps with the physical maps is also required for efficient localization and isolation of the genes, to study the organization and evolution of the genome, and as an initial step for efficient whole genome sequencing. Plans have been implemented for an international collaboration to develop integrated genetic and physical maps of the citrus genome, with an intention to lead to a full genomic sequence of citrus. Along with this will come the ability to understand genetic and metabolic control of all critical aspects of the traits of economic importance (Jain and Pryadarshan, 2009).Molecular Markers Molecular markers are very useful tools to help plant breeders in facing this and other issues: they allow the study of genetic variation, the search of new sources of agronomically important genes, the identification of cultivars and the efficient management of segregant generations during plant breeding programs through marker-assisted selection (Asins, 2002). A molecular marker is a genetic difference that may exist between two plants, visualized by means of biochemical methods and located at a certain chromosomal position. When the genotype for one or more marker loci is known for two plants, inferences can be made about the genotypes that may appear in the progeny. Isozyme analysis is the usual technique in the separation of zygotic from nucellar plants among progenies (Iglesias et al., 2009).Progress in the Citrus Genome Project coupled with recent advances in molecular biology techniques should aid the development of faster, targeted, and more efficient tools to expedite citrus breeding. For instance, DNA markers for traits associated with seedlessness, such as parthenocarpy, male and female sterility, and self-incompatibility, could be used as tools for directed breeding. These markers could be further used in map-based or candidate gene approaches for the cloning of genes that encode important traits, thus expanding the list of genes that can be transformed to induce seedlessness.
CONCLUSION
For consumers quality is a term that usually applied for the fresh fruit and within the markets refers to the external appearance of the product. Fruit color, fruit size, easy of peeling and seedlessness are paramount in commercial citrus types and new cultivars are being developed through plant breeding and selection of new sports. Therefore, conventional breeding of fruit trees will most likely continue to be a very important way to meet consumer demand for improved fruit quality. Triploidy is probably the most popular method for the development of seedless citrus cultivars. Fruit color is a unique characteristic in the life cycle of fruiting plants and is one of the most important traits for both commercial and esthetic value of fruit. Mutants are invaluable plant materials to help understand the relationship between genotype and phenotype and Mutation induction has arisen as a useful technique to increase genetic variability in citrus. Although citrus breeding is very challenging, breeding programmes throughout the world are making significant progress in the application of conventional and modern approaches to genetic improvement. Advances in plant cell and tissue culture also have major impacts on genetic improvement of citrus. The various methods for obtaining varieties with best fruit quality may be applied to different types of cultivars/varieties (sterile, mono or polyembryonic) and lead to very different populations in terms of genetic segregations and recombinations. Most of the critical goals for scion improvement, such as resistance to diseases or changes in fruit quality attributes, are difficult to approach in any practical sense by conventional breeding strategies. Rootstock improvements will also be hastened and maximized through the application of new knowledge and tools developed from genomic technology. The knowledge and establishment of genomics and bioinformatics have provided efficient tools for tagging and cloning of important genes. Biotechnology with different methods and strategy can be used to develop improved cultivars in many economically important citrus species.
REFERENCES
ABOUZARI, A. and FAKHERI, B. A. 2015. Reactive oxygen species: Generation, Oxidative Damage, and Signal Transduction. International Journal of Life Sciences, 9: 3–17.
AJIKUMAR, P., TYO, K., CARLSEN, S. et al. 2008. Terpenoids: Opportunities for biosynthesis of natural product drugs using engineered microorganisms. Molecular Pharmaceutics, 5: 167–190.
ALCOLEA, J. F., CANO, A., ACOSTA, M. and ARNAO, M. B. 2002. Hydrophilic and lipophilic antioxidant activities of grapes. Nahrung-Food, 46: 353–356.
ARAKAWA, O., HORI, Y. and OGATA, R. 1985. Relative eff ectiveness and interaction of ultraviolet-B, red and blue light in anthocyanin synthesis of apple fruit. Physiologia Plantarum, 64: 323–327.
ARTÉS, F., MÍNGUEZ, M. I. and HORNERO, D. 2002. Analysing changes in fruit pigments. In: MACDOUGALL, D. B. (ed.), Colour in Food: Improving Quality. Cambridge, UK: Woodhead Publishing Limited, 248–282.
ASINS, M. J. 2002. Present and future of quantitative trait locus analysis in plant breeding. Plant Breed, 121: 281–291.
BADENES, M. L. and BYRNE, D. H. (eds.). 2012. Fruit breeding (Vol. 8). Springer Science & Business Media.
BAYINDIRLI, A. 2010. Enzymes in fruit and vegetable processing: chemistry and engineering applications. CRC Press.
BROERTJES, C. (ed.). 2012. Application of mutation breeding methods in the improvement of vegetatively propagated crops (Vol. 2). Elsevier.
BROWN, A. G. 1975. Apples, Advances in Fruit Breeding. Purdue University Press, Lafayette.
BROWN, J. and CALIGARI, P. 2011. An introduction to plant breeding. John Wiley & Sons.
CAMERON, J. W. and FROST, H. B. 1968. Genetic, breeding and nucellar embryony. In: REUTHER, W., BATCHELOR, L. D. and WEBBER, H. J. (eds.), The citrus industry. Vol. 1. Riverside: Univ. California, 325–370.
CANNELL, M. G. and JACKSON, J. E. 1985. Attributes of trees as crop plants. Institute of Terrestrial Ecology.
CHEN, S., GAO, F. and ZHANG, J. 1991. Studies on the seedless character of citrus induced by irradiation. Mutation Breed. Newslet., 39: 8–9.
CHEN, C., LYON. M. T., O’MALLEY, D., FEDERICI, C. T., GMITTER, J., GROSSER, J. W., CHAPARRO, J. X., ROOSE, M. L., GMITTER JR., F. G. 2008. Origin and Frequency of 2n Gametes in Citrus sinensis × Poncirus trifoliata and Their Reciprocal Crosses. Plant Science, 174: 1–8.
COSTA, M. C. G., OTONI, W. C. and MOORE, G. A. 2002. An evaluation of factors aff ecting the effi ciency of Agrobacterium-mediated transformation of Citrus paradisi (macf.) and production of transgenic plants containing carotenoid biosynthetic genes. Plant Cell Rep., 21: 365–373.
DAVIES, F. S. and ALBRIGO, L. G. 1994. Fruit quality, harvesting and postharvest technology. In: Citrus. Wallingford Oxon UK: CAB International, 202–224.
DENG, Z. and XU, J. 2011. Breeding for fruit quality in citrus. In: Breeding for fruit quality, 349–371.
DONINI, B. 1982. Mutagens applied to improve fruit trees: Techniques, methods and evaluation of radiation induced mutations. In: Induced Mutations in Vegetatively Propagated Plants II. Vienna: IAEA, 29–36.
DUGO, G. and DI GIACOMO, A. (eds.). 2003. Citrus: the genus citrus. CRC Press.
FAO. 2015. Faostat-database. Available at: www.fao.org.FARAGHER, J. D. 1983.Temperature regulation of anthocyanin accumulation in apple skin. Journal of Experimental Botany, 34: 1291–1298.
FRONEMAN, I. J., BREEDT, H. J., KOEKEMOER, P. J. J. and VAN RENSBURG, P. J. J. 1996. Producing seedless Citrus cultivars with gamma irradiation. In: Proceedings of the Eighth International Citrus Congress. International Society of Citriculture. Sun City, South Africa, 1: 159–163.
GEORGE, W. L., SCOTT, J. W., SPLITTSTOESSER, W. E. 1984. Parthenocarpy in tomato. Horticultural Reviews, 6: 65–84.
GMITTER, F. G., JR., GROSSER, J. W. and MOORE, G. A. 1992. Citrus. In: HAMMERSCHLAG, F. A. and LITZ, R. E. (eds.), Biotechnology of perennial fruit crops. Wallingford: CABI, 335–369.
GMITTER, F. G., JR., XIAO, S. Y., HUANG, S., HU, X. L., GARNSEY, S. M. and DENG, Z. 1996. A localized linkage map of the citrus tristeza virus resistance gene region. Theor. Appl. Genet., 92: 688–695.
GROSSER, J. W., OLLITRAULT, P. and OLIVARES-FUSTER, O. 2000. Somatic hybridization in Citrus: an eff ective tool to facilitate cultivar improvement. In Vitro Cellular and Development Biology – Plant, 36: 434–449.
HEARN, C. J. 1984. Development of seedless orange, citrus sinensis, cultivar Pineapple and grapefruit, Citrus paradisi, cultivars through seed irradiation. J. Am. Soc. Hort. Sci., 109: 270–273.
HEARN, C. J. 1986. Development of seedless grapefruit cultivars through budwood irradiation. J. Am. Soc. Hort. Sci., 111: 304.HENSZ, R. A. 1971. Star Ruby, a new deep-red-fl eshed grapefruit variety with distinct tree characteristics. J. R io Grande Valley Hort. Soc., 25: 54–58.
HENSZ, R. A. 1977. Mutation breeding and the development of the Star Ruby grapefruit. In: Proc. Int. Soc. Citriculture. Orlando, FL, 2: 582.
HILLEBRAND, S., SCHWARZ, M. and WINTERHALTER, P. 2004. Characterization of anthocyanins and pyranoanthocyanins from blood orange (Citrus sinensis [L.] Osbeck) juice. Journal of Agricultural and Food Chemistry, 52: 7331–7338.
HOROWITZ, R. M. and GENTILI, B. 1977. Flavonoids constituen of citrus. In: NAGY, S., SHAW, P. E. and VELDUIS, M. K. (eds.). Citrus Science and Technology. Westport, CO, USA: AV1 Publ. Comp., Inc., I: 397–426.
IGLESIAS, D. J., COLMENERO-FLORES, J. M., IBÁÑEZ, V., BRUMOS, J., HERRERO-ORTEGA, M. A., RIOS, G., CERCOS, M. et al. 2009. Citrus genomics and breeding: identifi cation of candidate genes by the use of mutants and microarrays. In: II International Symposium on Citrus Biotechnology, 892: 19–25.
JACKSON, L. K. 1987. Research in the IFAS Fruit Crops Department. The Citrus Industry, 68: 2–4.
JAIN, S. M. and PRYADARSHAN, P. M. 2009. Breeding plantation tree crop: temperate species. Springer.
JANICK, J. 2008. Plant breeding reviews. John Wiley & Sons.
JENKS, M. A. and BEBELI, P. (eds.). 2011. Breeding for fruit quality. John Wiley & Sons.
JUÁREZ, J., ALEZA, P., OLIVARES-FUSTER, O. and NAVARRO, L. 2004. Recovery of tetraploid clementine plants (Citrus clementina hort. Ex Tan.) by in vitro colchicine treatment of shoot tips. Proc. Int. Soc. Citriculture, 1: 151–154.
KAYESH, E., SHANGGUAN, L., KORIR, N. K., SUN, X., BILKISH, N., ZHANG, Y. and FANG, J. 2013. Fruit skin color and the role of anthocyanin. Acta physiologiae plantarum, 35: 2879–2890.
KHAN, I. A. (ed.). 2007. Citrus genetics, breeding and biotechnology. CABI.
KOLTUNOW, A. M., VIVIAN-SMITH, A. and SYKES, S. R. 2000. Molecular and conventional breeding strategis for seedless citrus. Acta Hortic., 535: 169–174.
LADANYIA, M. and LADANIYA, M. 2010. Citrus fruit: biology, technology and evaluation. San Diego, California, USA: Academic press.
LATTANZIO, V. 2003. Bioactive polyphenols: Their role in quality and storability of fruit and vegetables. Journal of Applied Botany, 77: 128–146.
LEE, L. S. 1988. Citrus polyploidy-origins and potential for cultivar improvement. Crop and Pasture Science, 39: 735–747.
LEE, H. S. 2002. Characterization of major anthocyanins and the color of red-fl eshed budd blood orange (Citrus sinensis). Journal of Agricultural and Food Chemistry, 50: 1243–1246.
LE MARCHAND, L. 2002. Cancer preventive eff ects of fl avonoids—A review. Biomedicine and Pharmacotherapy, 56: 296–301.
LIN, S., GEORGE, W. L., SPLITTSTOESSER, W. E. 1984. Expression and inheritance of parthenocarpy in ‘Severianin’ tomato. Journal of Heredity, 75: 62–66.
LURO, F., MADDY, F., JACQUEMOND, C., FROELICHER, Y., MORILLON, R., RIST, D. and OLLITRAULT, P. 2004. Identifi cation and evaluation of diplogyny in clementine (Citrus clementina) for use in breeding. Acta Hort., 663: 841–847.
MATUS, J. T., AQUEA, F., ARCE-JOHNSON, P. 2008. Analysis of the grape MYB R2R3 subfamily reveals expanded wine quality-related clades and conserved gene structure organization across Vitis and Arabidopsis genomes. BMC Plant Biol., 8: 83.
NAVARRO, L. and JUAREZ, J. 2007. Shoot-tip gra ing in vitro: impact in the citrus industry and research applications. In: KHAN, I. A. (ed.), Citrus genetics, breeding and biotechnology. Wallingford: CAB International, 353–364.
NUEZ, F., COSTA, J., CUARTERO, J. 1986. Genetics of the parthenocarpy for tomato varieties ‘Sub-Arctic Plenty’, ‘75/59’ and ‘Severianin’. Z. P. Pfl anzenzuchtung, 96: 200–206.
OLLITRAULT, P., DAMBIER, D., LURO, F. and FROELICHER, Y. 2008. Ploidy manipulation for breeding seedless triploid citrus. Plant Breeding Reviews, 30: 323–352.
OTTO, S. P. and WHITTON, V. 2000. Polyploid incidence and evolution. Annu. Rev. Genet., 34: 401–437.
PICHA, D. 2006. Horticultural crop quality characteristics important in international trade. In: IV International Conference on Managing Quality in Chains – The Integrated View on Fruits and Vegetables Quality, 712: 423–426.
POMAR, F., NOVO, M., MASA, A. 2005. Varietal diff erences among the anthocyanin profi les of 50 red table grape cultivars studied by high performance liquid chromatography. J. Chromatogr., 109: 34–41.
PRETEL, M. T., BOTELLA, M. A., AMORÓS, A., ZAPATA, P. J. and SERRANO, M. 2007. Optimization of vacuum infusion and incubation time for enzymatic peeling of Thomson and Mollar oranges. LWT-Food Science and Technology, 40: 12–30.
RAMANA, K. V. R., GOVINDARAJAN, V. S., RANGANNA, S. and KEFFORD, J. F. 2009. Citrus fruits — varieties, chemistry, technology, and quality evaluation. Part I: Varieties, production, handling, and storage. C R C Critical Reviews in Food Science and Nutrition, 15: 353–431.
RAPISARDA, P., FABRONI, S., PETEREK, S. et al. 2009. Juice of new citrus hybrids (Citrus clementina Hort. ex Tan. × C. sinensis L. Osbeck) as a source of natural antioxidants. Food Chemistry, 117: 212–218.
RAY, P. K. 2002. Citrus. In: Breeding Tropical and Subtropical Fruits. Narosa Publishing House, 84–106.
RECUPERO, G. R., RUSSO, G. and RECUPERO, S. 2005. New promising Citrus triploid hybrids selected from crosses between monoembryonic diploid female and tetraploid male parents. HortScience, 40: 516–520.
ROBARDS, K. and ANTOLOVICH, M. 1997. Analytical chemistry of fruit bioflavonoids. A review. Analyst, 122: 11R–34R.
RODRIGUEZ-AMAYA, D. B. 1997. Carotenoids and FoodPreparation: The Retention of Provitamin A Carotenoids in Prepared, Processed and Stored Foods. Washington, D.C.: Agency for International Development, OMNI/USAID.
ROOSE, M. L. and WILLIAMS, E. 2007. Mutation Breeding. In: KHAN, I. A. (ed.), Citrus genetics, breeding and biotechnology. Wallingford: CAB International, 345–352.
SAUNT, J. 2000. Citrus varieties of the world. An illustrated guide. Norwich, England: Sinclair International limited.
SHEN, D. X. 1997. Fruit Breeding. 1st edition. Beijing: Agricultural Press.
SIMPSON, K. L. 1986. Chemical changes in natural food pigments. In: Chemical Changes in Food during Processing. Westport, CT: AVI, 409–441.
SINHA, N., SIDHU, J., BARTA, J., WU, J. and CANO, M. P. (eds.). 2012. Handbook of fruits and fruit processing. John Wiley & Sons.
SOOST, R. K . 1968. The incompatibility gene system in citrus. In: Proceeding of the First International Citrus Symposium. University of California. Riverside, California, 1: 189–190.
SOOST, R. K. and CAMERON, J. W. 1975. Citrus. In: JANICK, J. and MOORE, J. N. (eds.), Advances in Fruit Breeding. West Lafayette, Ind: Purdue University Press, 507–540.
SOOST, R. K. and ROOSE, M. L. 1996. Citrus. In: JANICK, J. and MOORE, J. N. (eds.), Fruit breeding, Vol. I: Tree and Tropical Fruits. New York. Chichester, Brisbane, Toronto, Singapore: John Wiley and Sons Inc., 257–323.
SPIEGEL-ROY, P., VARDI, A., ELHANATI, A. 1985. Seedless induced mutant in lemon (Citrus limon). Mutat. Breeding Newsl., 26.
SPIEGEL-ROY, P. 1990. Economic and agricultural impact of mutation breeding in fruit trees. Mutation Breeding Review, Vienna, 5: 215–235.
SPIEGEL-ROY, P., GOLDSCHMIDT, E. E. 1996. The biology of citrus. Cambridge University Press.
SPRINGOB, K., NAKAJIMA, J., YAMAZAKI, M. et al. 2003. Recent advances in the biosynthesis and accumulation of anthocyanins. Natural Product Reports, 20: 288–303.
STOVER, E., CASTLE, W. and CHAO, C. 2005. Trends in U.S. sweet orange, grapefruit, and mandarintype cultivars. Hort Technology, 15: 12–17.
SWINGLE, W. T. and REECE, P. C. 1967. The botany of Citrus and its wild relatives. In: WEBER, R. W. H. and BATCHELOR, L. (eds.), The Citrus Industry. 1. History, World Distribution, Botany and Varieties. Berkeley, USA: University of California Press, 190–430.
TADEO, F. R., CERCÓS, M., COLMENERO-FLORES, J. M., IGLESIAS, D. J., NARANJO, M. A., RÍOS, G. and TALON, M. 2008. Molecular physiology of development and quality of citrus. Advances in Botanical Research, 47: 147–223.
TALON, M., ZACARIAS, L., PRIMO-MILLO, E. 1992. Gibberellins and parthenocarpic ability in developing ovaries of seedless mandarins. Plant Physiology, 99: 1575–1581.
TANAKA, T. 1977. Fundamental discussion of Citrus classifi cation. Stud. Citrologia, 14: 1–6.
TON, L. D, KREZDORN, A. H. 1967. Growth of pollen tubes in three incompatible varieties of citrus. In: Proceedings of the American Society for Horticultural Science, 89: 211–215.
TORRES, D. C., DI’AZ-MAROTO, M. C., HERMOSI’N-GUTIE’RREZ, I., PE’REZ-COELLO, M. S. 2010. Effect of freeze-drying and oven-drying on volatiles and phenolics composition of grape skin. Anal. Chim. Acta, 660: 177–182.
TRIPOLI, E., LA GUARDIA, M., GIAMMANCO, S. et al. 2007. Citrus fl avonoids: Molecular structure, biological activity and nutritional properties: a review. Food Chemistry, 104: 466–479.
TUSA, N., FATTA DEL BOSCO, S., NARDI, L. and LUCRETTI, S. 1996. Obtaining triploid plants by crossing Citrus limon cv ‘Feminello’ 2n*4n Allotetraploid somatic hybrids. In: 8th Int. Cong. Citriculture. Int. Soc. Citr., South Africa, 1: 133–136.
UZUN, A., GULSEN, O., KAFA, G., SEDAY, U. 2008. ‘Alata’, ‘Gulsen’, and ‘Uzun seedless lemons and ‘Eylul early-maturing lemon. HorScience, 43(6): 1920–1921.
VARDI, A. and SPIEGEL-ROY, S. 1982. Plant regeneration from citrus protoplast: Variability in methodological requirements among cultivated species. Theoret. Appl. Genet., 62: 171–176.
VARDI, A., FRYDMAN SHANI, A. and WEINBAUM, S. A. 1988. Assessment of parthenocarpic tendency in citrus using irradiated marker pollen. Proc. Int. Soc. Citriculture, 1: 225–230.
VARDI, A., SPIEGEL-ROY, P. and ELCHANATI, A. 1993. Mandarin tree named Mor. US patent PP8, 378. USA.
VARDI, A., NEUMAN, H., FRYDMAN-SHANI, A., YANIV, Y., SPIEGEL-ROY, P. 2000. Tentative model on the inheritance of juvenility, self-incompatibility and parthenocarpy. Acta Horticulturae, 535: 199–205.
VITHANAGE, V. 1991. Eff ect of diff erent pollen parents on seediness and quality of ‘Ellendale’tangor. Scientia horticulturae, 48: 253–260.
WAKANA, A., HANADA, N., PARK, S. M., FUKUDOME, I. and KAJIWARA, K. 2005. Production of tetraploid forms of acid citrus cultivars by top gra ing of shoots with sprouting axially buds treated with colchicine. J. Fac. Agr. Kyushu Univ., 50: 93–102.
WHEELWRIGHT, N. T., JANSON, C. H. 1985. Colors of fruit displays of birddispersed plants in two tropical forests. Am. Nat., 126: 777–799.
WHITAKER, J. R. 1984. Pectic substances, pectic enzymes and haze formation in fruit juices. Enzyme and Microbial Technology, 6: 341–349.
YAMAMOTO, M., MATSUMOTO, R. and YAMADA, Y. 1995. Relationship between sterility and seedlessness in citrus. J. Japan. Soc. Hort. Sci., 64: 23–29.
The Investigation of Citrus Fruit Quality. Popular Characteristic and Breeding (PDF Download Available).
Available from: https://www.researchgate.net/publication/304813181_
The_Investigation_of_Citrus_Fruit_Quality_Popular_
Characteristic_and_Breeding [accessed Mar 21 2018].
No comments:
Post a Comment